Understanding limits on practical capacity of lithium iron phosphate batteries
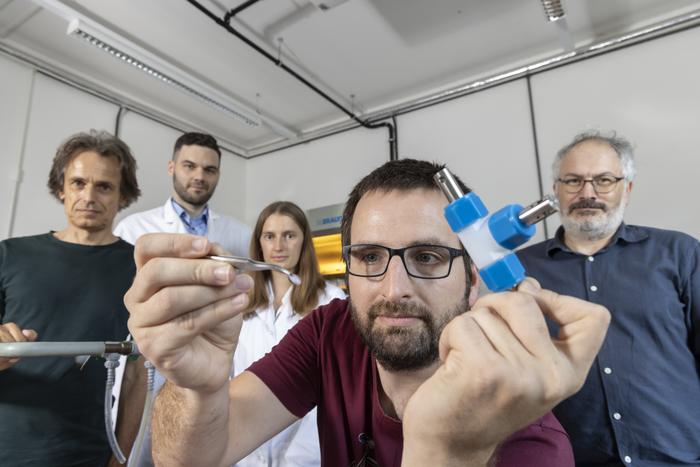
While significant research efforts have been made to explore its electrochemical kinetics in lithium iron phosphate batteries, debate persists regarding the mechanisms underlying the lithium insertion/extraction process and associated phase transitions, which largely determine the battery’s electrochemical performance. In particular, the origin of the large discrepancy between the theoretical capacity (170 mAhg−1) and the practical capacity, which is typically 10–25 % lower, remains a conundrum.
Now, using transmission electron microscopes, researchers at Graz University of Technology (TU Graz) were able to systematically track the lithium ions as they travelled through the battery material, map their arrangement in the crystal lattice of an iron phosphate cathode with unprecedented resolution and precisely quantify their distribution in the crystal.
“Our investigations have shown that even when the test battery cells are fully charged, lithium ions remain in the crystal lattice of the cathode instead of migrating to the anode. These immobile ions incur a cost in capacity,” says Daniel Knez from the Institute of Electron Microscopy and Nanoanalysis at TU Graz.
The immobile lithium ions are unevenly distributed in the cathode. The researchers have succeeded in precisely determining these areas of different levels of lithium enrichment and separating them from each other down to a few nanometres. Distortions and deformations were found in the crystal lattice of the cathode in the transition areas.
“These details provide important information on physical effects that have so far counteracted battery efficiency and which we can take into account in the further development of the materials,” says Ilie Hanzu from the Institute of Chemistry and Technology of Materials.
For their investigations, the researchers prepared material samples from the electrodes of charged and discharged batteries and analysed them under the atomic-resolution ASTEM microscope at TU Graz. They combined electron energy loss spectroscopy with electron diffraction measurements and atomic-level imaging.
“By combining different examination methods, we were able to determine where the lithium is positioned in the crystal channels and how it gets there,” explains Nikola from the Institute of Electron Microscopy and Nanoanalysis and first author of the paper.
According to Šimić, these newly-developed methods and the knowledge about ion diffusion can be transferred to other battery materials with only minor adjustments.
The research results were discussed in Phase Transitions and Ion Transport in Lithium Iron Phosphate by Atomic-Scale Analysis to Elucidate Insertion and Extraction Processes in Li-Ion Batteries published in Advanced Energy Materials.